Gut microbiome on immune checkpoint inhibitor therapy and consequent immune-related colitis: a review
Article information
Abstract
Immune checkpoint inhibitors have dramatically revolutionized the therapeutic landscape for patients with advanced malignancies. Recently, convincing evidence has shown meaningful influence of gut microbiome on human immune system. With the complex link between gut microbiome, host immunity and cancer, the variations in the gut microbiota may influence the efficacy of immune checkpoint inhibitors. Indeed, some bacterial species have been reported to be predictive for cancer outcome in patients treated with immune checkpoint inhibitors. Although immune checkpoint inhibitors are currently proven to be an effective anti-tumor treatment, they can induce a distinct form of toxicity, termed immune-related adverse events. Immune-related colitis is one of the common toxicities from immune checkpoint inhibitors, and it might preclude the cancer therapy in severe or refractory cases. The manipulation of gut microbiome by fecal microbiota transplantation or probiotics administration has been suggested as one of the methods to enhance anti-tumor effects and decrease the risk of immune-related colitis. Here we review the role of gut microbiome on immune checkpoint inhibitor therapy and consequent immune-related colitis to provide a new insight for better anti-cancer therapy.
INTRODUCTION
Cancer immunotherapy is an emerging field in cancer treatment [1]. The basis of cancer immunotherapy is to strengthen or activate immune response against tumor cells. Currently, immune checkpoint inhibitors (ICIs) are one of the most frequently used treatments in this field [1]. Immune checkpoints are involved in self-tolerance as a regulator of immune responses, and ICIs target immune checkpoints to enhance immune responses towards tumor cells, thereby exhibiting anti-tumor effects [1,2].
In 2011, the first ICI was approved by the U.S. Food and Drug Administration for the treatment of metastatic melanoma [1]. It was ipilimumab, a monoclonal antibody that inhibits cytotoxic T-lymphocyte-associated protein 4 (CTLA-4; CD152) [3]. Henceforth, ICI alone or ICI in combination with other cytotoxic agents have demonstrated effectiveness in treating various malignancies, including melanoma, non-small cell lung cancer (NSCLC), colorectal cancer, renal cell carcinoma (RCC), and gastric cancer [1,4]. Indications for the use of ICIs in cancer treatments are expected to grow rapidly in the future. Currently approved ICIs target either CTLA-4 or the programmed cell death protein 1 (PD-1)/programmed cell death-ligand 1 (PD-L1) axis. Examples of ICIs that target CTLA-4 include ipilimumab and tremelimumab, those that target PD-1 include nivolumab, pembrolizumab, and cemiplimab, and those that target PD-L1 include atezolizumab, durvalumab, and avelumab [1,5].
MECHANISMS OF ICIs
The T-cell receptor (TCR) is a protein complex on the surface of T cells that recognizes fragments of antigens presented by the major histocompatibility complex (MHC) on antigen-presenting cells (APCs) and other cells (Fig. 1) [1,6]. Likewise, CD28 is another protein located on the surface of T cells and is involved in co-stimulatory signals as well as in TCR-MHC signaling. CD28 binds to CD80 (B7-1) and CD86 (B7-2) expressed on the surface of APCs. Furthermore, CTLA-4 shares similar features with CD28 and binds to B7s with higher affinity than CD28 [7]. CTLA-4, combined with its ligand, degrades B7 through trans-endocytosis [8]. As a result, the co-stimulatory signal through CD28 decreases and T-cell activation is weakened. Through this mechanism, anti-CTLA-4 antibodies enhance T-cell activation by inhibiting CTLA-4, exhibiting anti-tumor effects.
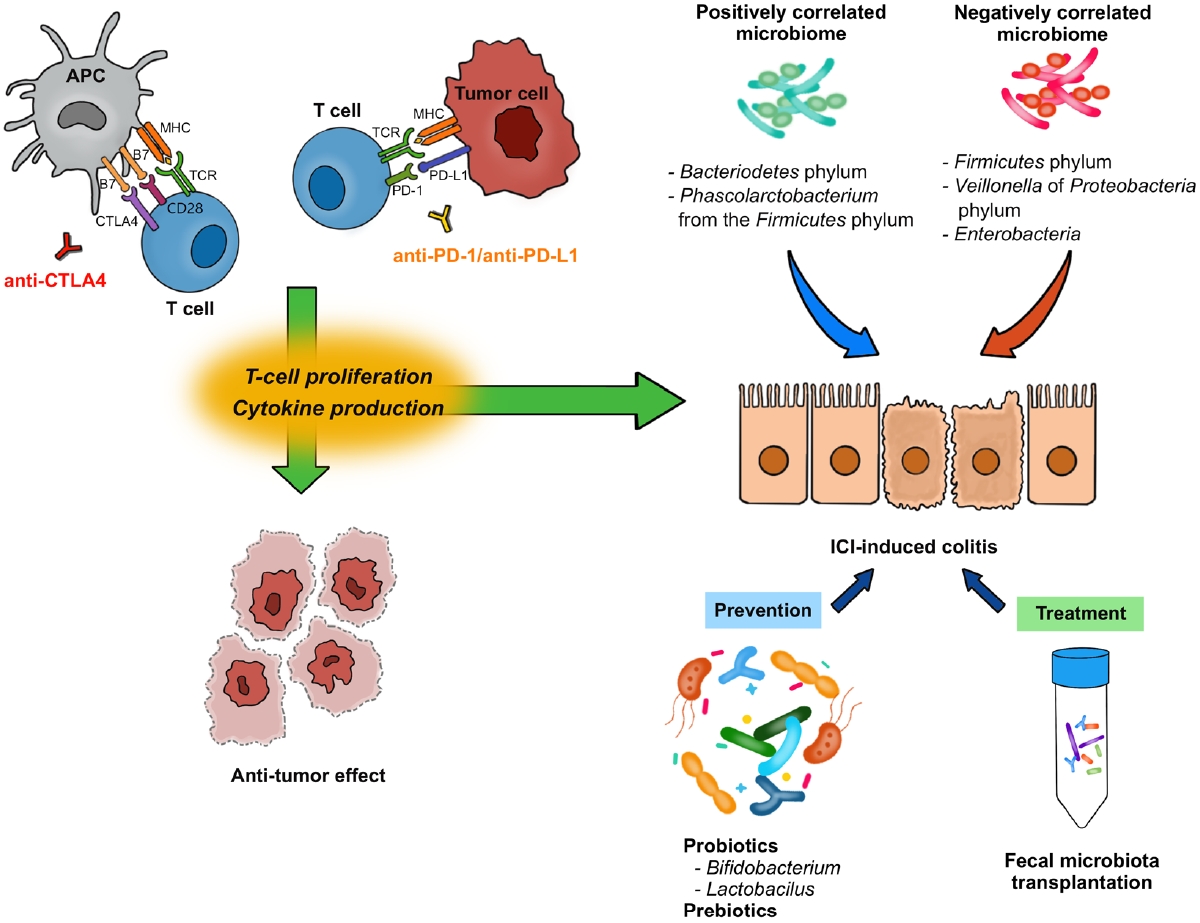
Mechanisms of immune checkpoint inhibitors (ICIs) and association of ICI-induced colitis with gut microbiome. APC, antigen-presenting cell; CTLA-4, cytotoxic T-lymphocyte-associated protein 4; PD-1, programmed cell death protein 1; PD-L1, programmed cell death-ligand 1; MHC, major histocompatibility complex; TCR, T cell receptor.
PD-1 (CD279) is a protein expressed on the surfaces of T cells and other immune cells. PD-1 ligands, including PD-L1 (B7-H1) and programmed cell death protein 2 (PD-L2; B7-H2), belong to the B7 family as well [9]. PD-L1 and PD-L2 are expressed in non-lymphoid tissues, including tumor cells, as well as APCs [9,10]. The PD-1/PD-L1 axis limits the T cell response in peripheral tissues, rather than in lymphoid organs. Downstream signals from PD-1 negatively regulate T-cell activation through the phosphorylation of TCR signaling intermediates [11]. PD-1 downstream signals also abrogate the intracellular signal from CD28, resulting in a dampened co-stimulatory effect of TCR-MHC signaling and reduced interleukin (IL)-2 production [10].
CTLA-4 inhibits the process by which APCs activate T cells, and the PD-1/PD-L1 axis inhibits the interaction between T cells and tumor cells. Through these processes, CTLA-4 and PD-1/PD-L1 axis contribute to both central and peripheral immune tolerances [10]. Monoclonal antibodies against CTLA-4, PD-1, and PD-L1 enhance immune responses against tumors and function as therapeutic agents against malignancies [1,9,10].
GUT MICROBIOME AND ANTI-TUMOR EFFECT OF ICIs
The gut microbiome includes all the microorganisms residing in the intestine and their metabolites. Not only does it affect intestinal homeostasis and inflammation, but it also influences carcinogenesis and the treatment of cancer [12,13]. Numerous studies on the gut microbiome and anti-tumor effect of ICIs have been reported in the last decade [22,23]. Most of these studies examined the relationship between the composition of the gut microbiome before and in response to ICI treatment (Table 1). Other studies have performed interventions to modulate the composition of the gut microbiome and analyzed the outcomes of such interventions [23].
Human studies on the relationship between ICIs and the gut microbiome tend to concentrate on melanoma, NSCLC, and RCC, which present early indications for ICI treatments [14-17,20,21,24]. Patients with melanoma with gut microbiomes enriched with Faecalibacterium genus and Firmicutes showed longer progression-free survival (PFS) and overall survival (OS) than those enriched with Bacteroides when treated with anti-CTLA-4 agents [14]. According to several studies examining the gut microbiome of patients with metastatic melanoma before anti-PD-1 therapy, those that responded to therapy had higher abundance of members of the Ruminococcaceae family, specifically Faecalibacterium, Bifidobacterium longum, Collinsella aerofaciens, and Enterococcus faecium, than non-responders [15,16,20]. Routy et al. [17] performed a metagenomic analysis of the fecal samples of 60 patients with NSCLC and 40 with RCC. Notably, Akkermansia muciniphila was enriched in patients with a favorable clinical response to anti-PD-1 therapy. Furthermore, oral supplementation of A. muciniphila to mice with non-responder feces-induced dysbiosis showed an enhanced PD-1 blockade effect. In the subgroup analysis of patients with NSCLC in this study, patients that responded to the therapy had enriched Ruminococcus, Alistipes, and Eubacterium compared to those that did not, whereas Bifidobacterium adolescentis, B. longum, and Parabacteroides distasonis were relatively less abundant [17].
MODULATION OF GUT MICROBIOME AND ITS IMPACT ON ANTI-TUMOR EFFECT OF ICIs
1. Fecal Microbiota Transplantation
Fecal microbiota transplantation (FMT) is a method that directly manipulates the recipient’s gut microbiome. Donor stool is transferred to the recipient’s gastrointestinal (GI) tract to induce therapeutic effects [25]. Pre-clinical studies with murine models reported the notable effectiveness of FMT [15-17]; therefore, phase 1 and 2 clinical trials on FMT for ICI-refractory cancer were conducted. According to Baruch et al. [18 10], patients with PD-1 refractory metastatic melanoma underwent FMT from 2 donors that responded to anti-PD-1 therapy, and 3 of the 10 patients showed a subsequent clinical response. In the subgroup consisting of 5 recipients with identical donors, the abundance of Enterococcaceae, Enterococcus, and Streptococcus australis was higher in responders than that in non-responders to the therapy. A phase 2 clinical study demonstrated that 6 out of 15 PD-1 refractory melanoma recipients showed a clinical response after FMT from long-term PD-1 responders. Enriched taxa in responders belonged to the phyla Firmicutes and Actinobacteria, whereas the phylum Bacteroidetes decreased in responders [19].
2. Probiotics
Probiotics are live microorganisms that can modify the bacteria composition in GI tract, subsequently providing beneficial effects to the human health, and they include a variety of bacteria such as Lactobacillus and Bifidobacterium [26]. They are widely used in treating various GI disease including inflammatory bowel disease (IBD), although the scientific evidence supporting the administration of probiotics is insufficient. There are studies examining the association between the use of probiotics and the anti-tumor effects of ICIs. According to a study by Spencer et al. [20], probiotic intakea had no effect on the survival outcomes of 158 patients with melanoma treated with ICI therapy. In contrast, patients with RCC orally supplemented with bifidogenic live bacterial products and treated with dual ICI (nivolumab and ipilimumab) therapy showed longer PFS than those that remained unsupplemented [21]. Notable human-based studies reporting on the modulation of gut microbiome by FMT or probiotics and its anti-tumor effects are summarized in Table 1.
3. Antibiotics
Antibiotic use is common in patients with tumors due to comorbid infections. Studies examining the relationship between antibiotic usage and ICI efficacy have shown relatively consistent results [27-30]. According to a landscape prospective study involving 196 patients, prior usage of antibiotics up to 30 days before ICI therapy resulted in worse OS and response rate to anti-PD-1/PD-L1 therapy [27]. Primarily beta-lactam antibiotics were investigated, and when the antibiotics and ICIs were used concurrently, the reduced efficacy of ICIs was not observed. This study indicates that prior use of broad-spectrum antibiotics may cause gut dysbiosis and subsequently reduce ICI efficacy, whereas their simultaneous administration may remain harmless [27]. Ahmed et al. [28] reported inferior PFS and a lower response rate in patients treated with antibiotics, with worse results observed in patients treated with broad-spectrum antibiotics. Other studies have also reported detrimental effects of prior antibiotic use on ICIs. Prior antibiotic usage resulted in shorter PFS and OS in patients with NSCLC/RCC receiving anti-PD-L1 therapy [29] and shorter PFS and lower response rate in patients with melanoma receiving ICI therapy [30]. In summary, the use of antibiotics before ICI treatment causes gut microbial dysbiosis, weakening the anti-tumor effects of ICIs.
Despite these results on the relationships between FMT, probiotics, antibiotics, and efficacy of ICIs, there is still much to be investigated concerning the mechanisms by which the gut microbiome affects the anti-tumor effect of ICI.
UNDERLYING MECHANISMS OF GUT MICROBIOME ON ANTI-TUMOR EFFECTS OF ICIs
Regulating both innate and adaptive immunity, gut microbiome exhibits a significant influence on the human immune system. Pattern-recognition receptors (PRR) at the intestinal epithelial cells are activated by gut microbiome, and their downstream signaling leads to regulation of antimicrobial response with the expression of pro-inflammatory cytokines such as tumor necrosis factor, IL-1, and IL-6 [13,31]. This process of activated innate immunity by gut microbiome and PRR subsequently leads to activation of adaptive immunity. Oral administration of the probiotic Lactobacillus rhamnosus GG augmented the anti-tumor activity of anti-PD-1 in a murine model. Si et al. [32] explained this result as the activation of dendritic cells by L. rhamnosus GG and subsequent type 1 interferon production, resulting in the enhanced cross-priming of anti-tumor CD8+ T cells. A mouse model by Bessel et al. [33] showed that antigen-specific T cells cross-react with commensal Bifidobacterium breve and tumor antigens, indicating that commensal bacteria can stimulate an immune response against tumors via cross-reactivity. According to Overacre-Delgoffe et al. [34], colonization of Helicobacter hepaticus in a mouse model of colorectal cancer induces H. hepaticus-specific T follicular helper cells, which in turn mature tertiary lymphoid structures adjacent to the tumor. This indicates that microbiome-reactive CD4+ T cells exhibit anti-tumor activity. These studies demonstrate how the gut microbiome modulates the immune response against tumors and can influence the anti-tumor effect of ICIs.
Short-chain fatty acids (SCFAs) are major metabolites produced by the gut microbiome in the colon. Nomura et al. [35] performed a prospective study on fecal and serum SCFA concentrations and their changes in response to anti-PD-1 antibodies. The results showed that higher SCFA levels (fecal acetic acid, propionic acid, butyric acid, valeric acid, and plasma isovaleric acid) were correlated with longer PFS. In another study, the gut microbiome of patients with NSCLC responsive to anti-PD-1 therapy was associated with the production of SCFAs [36]. SCFAs can directly promote differentiation of both effector and regulatory T cells under different immunologic conditions, depending on the presence of various cytokines [37]. Bifidobacterium pseudolongum enhances the response to ICIs through the production of the metabolite inosine. Systemic translocation of inosine activates anti-tumor T cells, an effect that is dependent on T-cell expression of the adenosine A2A receptor [38].
In summary, gut microbiome seems to influence the immune system through several mechanisms. It activates PRR at the intestinal epithelial cells, which subsequently impacts both innate and adaptive immune response. Also, gut microbiome affects T cells through both cross-reactivity and direct involvement by its metabolites. These mechanisms operate in a coordinated manner, underscoring the importance of the gut microbiome’s initial composition and modulation in determining the efficacy of ICI. Nevertheless, the intricate mechanisms between the gut microbiome and the anti-tumor effects of ICIs remain to be fully understood, warranting further research.
IMMUNE-RELATED ADVERSE EVENTS ASSOCIATED WITH ICIs
With an increase in immune system activity, ICIs can induce inflammatory side effects (i.e., immune-related adverse events) [39]. Theoretically, any organ system can be affected by increased immune activity; the skin, GI tract, liver, and endocrine glands are most often involved [39,40]. The cardiovascular, pulmonary, central nervous, musculoskeletal, and hematologic systems are less frequently involved. The variations in the incidence of immune-related adverse events may be largely influenced by the type of ICI, nature of the cancer, organs involved, and study design [41].
Although several mechanisms have been suggested in the literature, the precise mechanism underlying ICI-induced immune-related adverse events remains unclear [39,42]. CTLA-4 and PD-1/PD-L1 inhibitors increase T-cell activation and proliferation and abrogate regulatory T cell functions [42]. Adult mice with a conditional deletion of the gene encoding CTLA-4 developed spontaneous lymphoproliferation, hypergammaglobulinemia, and multi-organ autoimmune diseases such as pneumonitis, gastritis, insulitis, and sialadenitis [43]. Two genetic diseases with shared mechanisms of autoimmunity in the CTLA-4 pathways, CHAI (CTLA-4 haploinsufficiency with autoimmune infiltration) and LATAIE (LRBA deficiency with autoantibodies, regulatory T cell defects, autoimmune infiltration, and enteropathy), have also been reported in humans [44]. CTLA-4 and PD-1/PD-L1 axis inhibitors also induce cytokine production. CD4+ and CD8+ T-cell activation, with subsequent release of cytokines such as tumor necrosis factor, interferon-γ, IL-2, and IL-17, have been reported in both experimental and human studies [42,45,46]. Cross-reactivity between anti-tumor T cells and T cells against similar antigens on normal cells might influence the development of some immune-related adverse events [39,42]. Increasing levels of autoantibodies may also be involved in the pathogenesis of immune-related adverse events [39]. The increased activity of T cells by ICIs can augment T cell–B cell interactions, consequently inducing autoantibody production. For example, anti-PD-1 or anti-PD-L1 treatment may enhance antithyroid antibodies in patients developing thyroiditis [47]. Other autoantibodies produced in response to ICI therapy have been identified, including autoantibodies to thyrotropin, follicle-stimulating hormone, and corticotropin-secreting cells in patients developing hypophysitis; rheumatoid factor and anti-cyclic citrullinated peptide in patients with arthritis; and diabetic autoantigens in patients with type 1 diabetes mellitus [42].
ICI-INDUCED COLITIS
Among the immune-related adverse events of ICI therapy, those of the GI tract are the most common and severe events [41]. Of the GI adverse events, ICI-induced colitis is the most frequently reported [48,49]. This immune-related adverse event, ranging from mild diarrhea to severe colitis, is most commonly reported 6 to 8 weeks after the first ICI infusion, although it may occur later or after discontinuation [48-50].
The most common practice for diagnosing ICI-induced colitis is a colonoscopic evaluation with biopsy and should be considered in patients with symptoms greater than grade 2 [50,51]. Colonoscopic evaluations can also be considered in patients with persistent grade 1 diarrhea despite conservative management or high levels of fecal inflammatory markers [50]. Common endoscopic findings include patchy or diffuse erythema, edema, friability, loss of vascularity, erosions, and ulcerations [52]. Even in patients with mucosa that appears normal, colonoscopic biopsies should be obtained because microscopic patterns can be observed in ICI-induced colitis [52]. Histological findings of ICI-induced colitis are not unique and share the characteristics of infectious colitis, microscopic colitis, and IBD [51]. Although cross-sectional imaging by computed tomography or magnetic resonance imaging has a limited role in diagnosing ICI-induced colitis, it may be useful in patients with severe symptoms (fever, severe pain) to rule out serious complications, such as perforation [50]. In a recent prospective study by Sakurai et al. [53], the ultrasonographic findings of ICI-induced colitis were determined along with the colonoscopic index. The authors indicated that these findings were primarily similar to those of ulcerative colitis and that ultrasonography could be useful for noninvasive monitoring of patients with ICI-induced colitis. The mainstay of treatment regimen for ICI-induced colitis consists of corticosteroids, budesonide, 5-aminosalicylic acids, or supportive care including anti-diarrheal agents [50]. Biologics such as infliximab and vedolizumab are known to be effective for second-line immunosuppression [50,54].
GUT MICROBIOME AND ICI-INDUCED COLITIS
From the experience of studies on IBD, GI immune-related adverse events are expected to be related with the gut microbiome [55,56]. Indeed, anti-tumor effects and colitis do not occur in germ-free mice, which suggests the effects of ICI rely on the gut microbiota [55,57]. In human, the development of ICI-induced colitis was reported to be associated with decreased diversity and a shift in composition of gut microbiota (Table 2) [16,58-60]. In patients with melanoma following anti-CTLA-4 treatment, Firmicutes (e.g., Faecalibacterium prausnitzii and Gemmiger formicilis) was associated with the occurrence of ICI-induced colitis, while Bacteroidetes was associated with no occurrence of colitis [16]. Dubin et al. [58] investigated baseline microbiota from metastatic melanoma patients prior to anti-CTLA-4 treatment and showed that a higher abundance of Bacteroidetes phylum and 3 of its families (Bacteroidaceae, Rikenellaceae, and Barnesiellaceae) was correlated with resistance to ICI-induced colitis. Liu et al. [59] explored the baseline microbiota of NSCLC patients treated with anti-PD-1 treatment. A higher abundance of Bacteroidetes phylum and lower abundance of Firmicutes phylum were demonstrated in diarrhea-free patients. While Veillonella of Proteobacteria phylum was lower, Bacteroides and Parabacteroides belonging to Bacteroidetes phylum and Phascolarctobacterium of Firmicutes phylum were higher in diarrhea-free patients [59]. Sakai et al. [60] reported, in patients with solid cancers following anti-PD-1 treatment, a decreased abundance of Bacteroides species in colonic mucosa sample obtained from patients who developed ICI-induced colitis. These results suggest that it might be possible to predict the risk of ICI-induced colitis based on the composition of gut microbiota before ICI treatment, although the current evidence is inconsistent [61].
GUT MICROBIOME IN MODULATING ICIINDUCED COLITIS
1. Probiotics
Regarding ICI-induced colitis, probiotics administration might be an effective method to reducing the risk. Wang et al. [62] first explore the protective role of Bifidobacterium in the mice model with anti-CTLA-4 antibody. After pretreatment with vancomycin, the colitis became more severe suggesting that Grampositive bacteria appear to have a mitigating effect. Conversely, Gram-positive probiotic Bifidobacterium significantly reduced the colitis in the mice model without an apparent effect on the anti-tumor immunity of ICI. Interestingly, the effects appeared to be dependent on regulatory T cells [62,63]. In the subsequent animal study with anti-CTLA-4 and anti-PD-1 antibodies, an obvious reduction in the abundance of Lactobacillus reuteri was noted in the ICI-induced colitis group [64]. Oral administration of L. reuteri ameliorated the colitis induced by ICI in the mice model, and this protective effect was associated with a decrease in the population of group 3 innate lymphocytes induced by ICI-induced colitis.64 Prebiotics, such as dietary fiber, also may promote growth of beneficial microbiota and production of their metabolites such as SCFA. They are generally accepted as a safe supplement to alter the microbiota [65]. Currently there is no clear evidence that the administration of prebiotics protects ICI-induced colitis. However, there is a possibility that prebiotics might play a protective role in ICI-induced colitis with the findings of the immunoregulatory role of SCFA which could induce colonic Treg proliferation [65,66].
2. Fecal Microbiota Transplantation
With the hypothesis that alteration of gut microbiota could be a promising way to effectively treat immune-mediated GI disease, FMT also has been suggested for the treatment of ICI-induced colitis, especially refractory cases resistant to corticosteroids and biologics [67,68]. Wang et al. [67] first reported 2 cases of refractory ICI-induced colitis which were successfully managed by FMT. After FMT, the reduced density of CD8+ T cells by increasing regulatory T density was noted in the colonic mucosa, with the beneficial enrichment of Bifidobacterium and Akkermansia [67]. Fasanello et al. [68] also reported a case of severe refractory ICI-induced colitis notably improved by FMT. The recent guidelines included FMT as an optional treatment for refractory ICI-induced colitis [50,69], although further investigation of FMT for the management of ICI-induced colitis is warranted.
CONCLUSION
The gut microbiome is known to be associated with the antitumor effects of ICIs and their immune-related adverse events. Therefore, many studies are in progress to explore specific alterations of the gut microbiota as biomarkers for ICI effects and immune-related adverse events. In addition, manipulation of the gut microbiome by FMT or probiotics administration has been suggested as a complementary method to improve the anti-tumor effects of ICIs and prevent immune-related adverse events. Although significant progress has been made regarding the role of the gut microbiome in ICI therapy, many issues remain to be explored in the future.
Notes
Funding Source
This work was supported by a grant from the Korean Gastroenterology Fund for Future Development and a grant (2022IP0079, 2023IP0058) from the Asan Institute for Life Sciences, Asan Medical Center, Seoul, Korea.
Conflict of Interest
No potential conflict of interest relevant to this article was reported.
Data Availability Statement
Not applicable.
Author Contributions
Conceptualization: Hwang SW, Kweon MN. Writing the original draft: Hwang SW, Kim MK. Writing review and editing: Hwang SW. Supervision: Hwang SW, Kweon MN. Approval of final manuscripts: all authors.
Additional Contributions
We wish to thank Dr. Hye Young Woo (Department of Surgery, Seoul National University Hospital, Seoul, Korea) for aiding the creation of the figure.